As native starch is only slowly degradable by the use of α-amylases, gelatinization and liquefaction of a 30-40% dry matter suspension is needed to make the starch susceptible to enzymatic breakdown. The gelatinization temperature is different for different starches (Lii and Chang, 1991). Maize starch is the most widespread source followed by wheat, tapioca and potato. A good liquefaction system is necessary for successful saccharification and isomerization. For liquefaction, an efficient temperature stable α-amylase is added to the starch milk. The mechanical method of performing the liquefaction may be by use of stirred tank reactors, continuous stirred tank reactors (CSTR) or a jet cooker.
In most plants, the starch liquefaction is made in a single-dose jet-cooking process as shown in Figure 2.2. The α-amylase Termamyl® is metered into the starch slurry after pH adjustment, and the slurry is pumped through a jet cooker. Live steam is injected here to raise the temperature to 105°C, and subsequent passage through a series of holding tubes provides a 5 min residence time, which is sufficient to fully gelatinize the starch. By flashing the partially liquefied starch the temperature is reduced to 90-100°C and the enzyme is allowed to react further at this temperature for 1-2 h until the required DE is obtained.
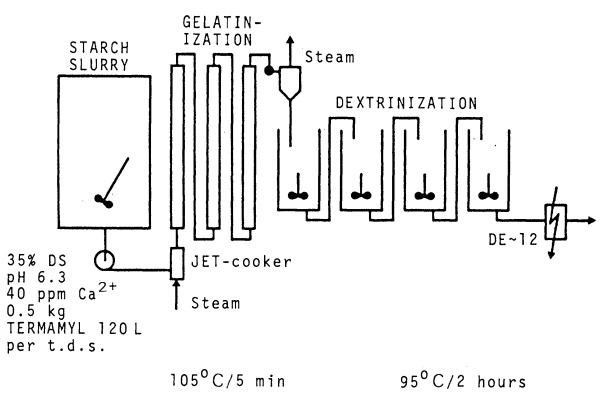
The jet-cooking process is normally carried out under the following conditions. Dry substance (DS) 30-35% (w/w) , pH 6.0-6.5 and a Ca2+ content of 20-80 ppm (w/v). The dose is typically between 0.5 and 0.6 kg Termamyl 120L per ton of starch (DS). The stability depends on temperature, Ca2+ concentration, ion content and DE. Rosendal et ai. (1979) described stability data from pilot plant trials, from where it was possible to estimate optimum design parameters for the use of Termamyl in individual processes, thus minimizing the dosage of enzymes. The relationship between half-life (T1/2) and the temperature was derived from an Arrhenius-type equation (plot of half-life versus the reciprocal absolute temperature). It was assumed that the stability under other conditions could be expressed as the standard half-life multiplied by correction factors for each parameter:
T1/2 = T1/2* x K1(pH) x K2(DS) x K3(Ca) x K4(μΩ)
From a practical point of view, stabilities of Termamyl (expressed in half-lives) are a function of temperature, dry substance (DS), pH, and calcium concentration (Table 2.2).
Reaction parameters | Half-life (min) | |
Temperature (oC) | 110 105 100 95 | 15 50 200 700 |
Dry substance (% w/w) | 35 30 25 | 50 30 20 |
pH | 6.5 6.0 5.5 | 200 110 40 |
Calcium concentration (ppm Ca2+) | 70 40 10 | 200 110 40 |
From Table 2.2 it is possible to deduce the enzyme stability for nearly any set of conditions. The values for the stabilities shown under ‘temperature’ are simply corrected for changes in DS, pH, and Ca2+ in the proportions found under the respective headings. For example, the stability of Termamyl at 105°C, 30% DS, pH = 6.0 and 10 ppm Ca2+ can be calculated to be:
50 X 30/50 X 110/200 x 15/50 = 5 min half life
The addition of Ca2+ up to a total of 40 ppm will increase the half-life to 16 min.
The enzyme activity decay follows a first-order reaction scheme according to the formula:
At = A0 x exp – (ln2:T1/2) x t
where
- At = the residual activity at time t,
- A0 = the activity at time 0,
- T1/2 = the enzyme stability (half-life) (min),
- t = the reaction time (min).
Hence, the residual activity after 5 min of reaction at the conditions used in the above example (half-life = 16 min):
A5 = 100 x exp [(-ln2/16) x 5] = 80%
In general, liquefaction is carried out at pH 6.0-6.5. Lower pH values may be used in such cases, where improved stability can be obtained by other means, e.g. by a reduced reaction temperature. Higher pH values should be avoided due to increased byproduct formation (e.g. maltulose precursors). Maltulose formation is enhanced at high temperatures, high pH and long residence times, and is more pronounced at increasing DEs. One advantage of Termamyl is that the temperature is increased only to 105°C at a very low DE compared with hydrochloric acid (at pH 1.8-2), where a temperature of 140°C is used to obtain DE 7-8. A B. subtilis α-amylase treatment at 85°C is sometimes used for thinning of the starch before pressure cooking with acid.
The above-mentioned process (Figure 2.2) can be used for the production of maltodextrins (DE 15-20). In this case, a higher enzyme dose and/or a longer residence time is needed.
Without too much success, cooking extruders have also been studied for the liquefaction of starch but due to the high temperature in the extruder, inactivation of the enzyme demands 5-10 times higher dosage levels than in a jet cooker (Chouvel et al., 1983).