Gari is a cassava semolina traditionally processed and widely consumed in West Africa. Its shelf-life is largely dependent on both its water content and storage temperature and relative humidity. This paper deals with gari’s adsorption properties in relation to water availability at 15, 25, and 30 °C. From the results, optimal packaging conditions can be estimated, thus providing an extended shelf-life for gari.
Introduction
Gari is a cassava semolina traditionally prepared and widely consumed in West Africa. Gari processing consists of pressing the juice out of peeled and grated cassava roots for 2 to 4 days, allowing a natural lactic fermentation to take place. The fermented mash is then cooked in an open clay vessel until the starch gelatinizes sufficiently (Chuzel, 1989; Zakhia, 1985). Gari is a “ready-to-use” food, generally consumed with milk (for breakfast) or added to hot sauces.
As demand for gari from urban markets is increasing, a better quality product with an extended shelf-life is needed. The shelf-life of packed and stored gari largely depends on storage temperature and relative humidity, and on the product itself (moisture content and water activity). These parameters determine the rate of gari’s microbial and physicochemical deterioration. The sorption isotherm, that is, the equilibrium between water activity and moisture content of a foodstuff, is a good indicator of the product’s stability at different ambient temperatures and relative humidities (Bandyopadhyay et al., 1980).
Our study used adsorption isotherms to determine gari’s optimal shelf-life. Thus, we could propose a set of packaging recommendations to ensure better storage of gari in the tropics.
Materials and Methods
Samples of traditional Togolese gari (about 8% w.b. water content) were collected from a small factory near Lomé and packaged in sealed polyethylene bags. They were sent to Montpellier, France, and stored at 2 °C until evaluation. Adsorption isotherms were determined at 15, 25, and 35 °C, using the standard method recommended by the Water Activity Group (WAG) of the European Union (EU)1 (Wolf et al., 1985). Equipment used comprised nine, sealed, glass jars, containing saturated salt solutions ranging from aw 0.1 to 0.9 (Table 1). This aw range is required practice for predicting the shelf-life of dried packaged products (Chirife et al., 1979).
In France, before measurements were taken, 3 g of gari samples were predried over phosphorus pentoxide (P2O5) for 10 days at about 20 °C (ambient temperature) to lower their water content to a minimum. They were then sprayed with a solution of sodium azide (0.5%) to inhibit the growth of microorganisms at high water activities (aw > 0.8). They were placed in dishes resting on trivets standing in jars of salt solutions. The sealed jars were then submerged in an insulated water bath, the temperature of which was controlled to within ± 0.2 °C.
Equilibrium time was 21 days, as recommended by WAG’s standard (i.e., 7 days for aw < 0.6 and 14 days for aw > 0.6), and in accord with the equilibration time required for cassava mash (Gevaudan et al., 1989). Equilibrated samples were oven-dried (103 °C, 24 h) in triplicate to determine moisture content.
The WAG uses the GAB (Guggenheim-Anderson-De Boer) model, which is believed to be the best for providing equations for describing food isotherms up to aw 0.9 (Bizot, 1983; Van den Berg, 1985). The GAB equation is as follows (Labuza et al., 1985):
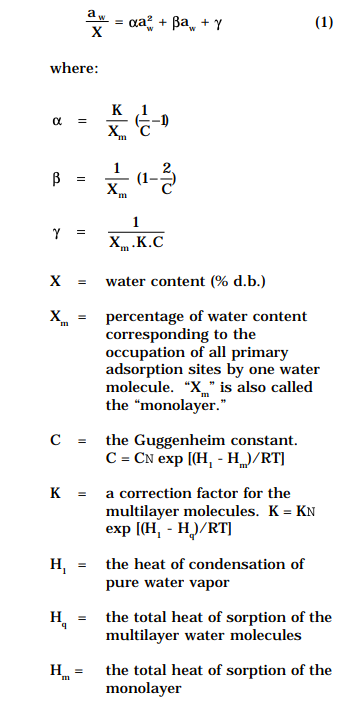
The GAB model’s coefficients α, β, and γ were determined for each temperature by using a nonlinear, least-square regression as recommended by Schär and Rüegg (1985). The values of GAB constants Xm, C, and K were also calculated. The confidence of fit was judged by the relative root mean square error (% RMS).
Results and Discussion
Adsorption isotherms
Table 1 and Figure 1 show the average values and standard deviations of the equilibrium moisture contents for the water activities studied at 15, 25, and 35 °C, and their fitted GAB isotherms.
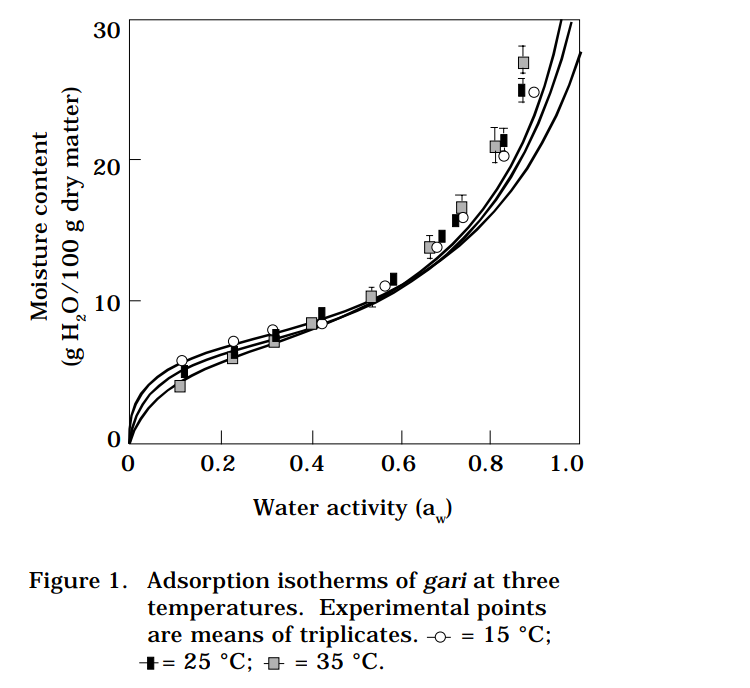
Salt | 15 °C | 25 °C | 35 °C | |||
Equilibrium relative humidity | Moisture content (% d.b. ± SD) | Equilibrium relative humiditya | Moisture content (% d.b. ± SD) | Equilibrium relative humidity | Moisture content (% d.b. ± SD) | |
LiCl | 0.119b | 5.2 ± 0.4 | 0.110 | 4.8 ± 0.1 | 0.112b | 4.4 ± 0.3 |
CH3COOK | 0.234c | 7.4 ± 0.4 | 0.224 | 6.7 ± 0.3 | 0.230d | 5.9 ± 0.2 |
MgCl2 | 0.333b | 8.1 ± 0.4 | 0.330 | 7.6 ± 0.2 | 0.320b | 7.3 ± 0.3 |
K2CO3 | 0.431c | 9.1 ± 0.2 | 0.428 | 8.6 ± 0.3 | 0.410e | 8.6 ± 0.2 |
NaBr | 0.607c | 11.4 ± 0.7 | 0.577 | 11.0 ± 0.2 | 0.545c | 10.3 ± 0.6 |
SrCl2 | 0.741b | 15.2 ± 0.8 | 0.708 | 14.3 ± 0.2 | 0.680d | 13.7 ± 0.8 |
NaCl | 0.755c | 15.4 ± 0.5 | 0.753 | 16.1 ± 0.2 | 0.751f | 16.6 ± 0.7 |
KCl | 0.856b | 20.7 ± 0.9 | 0.843 | 20.3 ± 0.6 | 0.829c | 20.8 ± 1.2 |
BaCl2 | 0.911b | 24.5 ± 0.8 | 0.902 | 26.7 ± 0.6 | 0.894g | 26.8 ± 1.2 |
a. Stokes and Robinson, 1949.
b. Acheson, 1965.
c. Greenspan, 1977.
d. Rockland, 1960.
e. Griffin, 1944.
f. Clarke and Glew, 1985.
g. Baxter and Cooper, 1924.
At low water activities (aw < 0.6), the experimental curves agree with the sorption theory, that is, at constant aw, an increase of temperature causes a small decrease of moisture content. At high water activities (0.7-0.9), moisture content increases with rise in temperature. The three calculated isotherms intersect at the following points:
isotherms 15 °C and 25 °C
where X = 13.0% and aw = 0.66
isotherms 15 °C and 35 °C
where X = 11.5% and aw = 0.59
isotherms 25 °C and 35 °C
where X = 9.5% and aw = 0.48
The crossing of isotherms at high water activities with increasing temperature has already been observed in some foods and may result from the product’s chemical composition and its treatments (e.g., heating, drying, and pregelatinization). Other foods showing this phenomenon are sucrose and fructose (Loncin et al., 1968), potato slices (Mazza, 1982), carrots (Mazza, 1983), Jerusalem artichoke (Mazza, 1984), and sultanas (Saravacos et al., 1986). The explanation is that some sugars increase their solubility with temperature, thus binding more water at higher temperatures and increasing the equilibrium moisture content.
The technological treatments involved in gari production (grating, fermentation, and squeezing) induce damage to about 3% to 6% of the cassava starch (Zakhia, 1985). During roasting, the starch is heated in the presence of water, but the initial moisture content of about 1 g g-1 (d.b.) of cassava mash does not allow the starch to completely gelatinize (Chuzel, 1989; Gevaudan et al., 1989). But crystallinity is lost and extensive swelling of the starch granules occurs. A complex metastable network forms, consisting of amorphous regions (containing plasticizing water) and hydrated microcrystalline regions that had not dissolved during the partial gelatinization and which serve as junction zones (Levine and Slade, 1988). All these factors strongly affect the polymer-water interactions (Radosta et al., 1989).
The sorption mechanism for starch is almost entirely governed by active sites, that is, the glucose residues of the starch polymer (Hill and Rizvi, 1982). We suggest, therefore, that increases in both temperature (to 35 °C) and water content (for aw between 0.5 and 0.7) initiate a “collapse” that makes the soluble starch (amorphous fractions and branched segments) leach out. This increases the number of available adsorption sites (glucose residues) and explains why gari becomes more hygroscopic at higher temperatures and water activity. Moreover, the degree of starch damage in gelatinized starchy products is measured by the solubility and swelling indices at 30 °C, which depend on the ability of starch to absorb water (Anderson et al., 1969).
Slade and Levine (1988) and Orford et al. (1989) have also discussed the physicochemical effect of water, acting as a plasticizer of the amorphous regions in the starch native granule, on the temperature of the vitreous transition that occurs during native starch gelatinization. Only the water included in the starch granule (about 10% w.b.) is involved in this process; the vitreous transition temperature decreases sharply with increasing water content. This plasticizing effect could also explain the observed adsorption behavior of gari. The amorphous matrix of gari, which is partially plasticized at room temperature by excess water (> 12% d.b.), would become more plastic if the temperature increased to 35 °C. The mobility of chains is then enhanced and the free volumes inside the polymer increase. As these free volumes may absorb more water, the sorption sites are then more available.
Gari storage
In tropical countries, high relative humidity and temperature make long-term storage very difficult. So predicting the shelf-life of packaged gari in terms of its storage conditions and quality becomes important. The Heiss and Eichner (1971a; 1971b) model allows calculation of the potential storage time based on a critical aw for a particular system under given storage conditions. This model equation is:
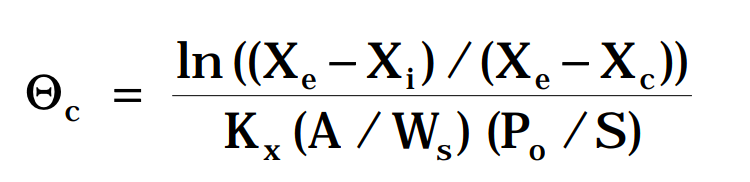
where:
- Θc = Potential shelf-life of the product (time in days for the packaged product to suffer microbial and biochemical deterioration with loss of sensory quality).
- Xe = Equilibrium moisture content (g g-1, d.b.) of the product (if it is left in contact with the atmosphere outside the package). “Xe ” depends on temperature, relative humidity and on the product adsorption isotherm.
- Xc = Safe storage moisture content of the product (g g-1, d.b.), that is, the moisture content corresponding to the safe storage borderline aw. “Xc ” is calculated for the borderline aw by successive iterations from the GAB regression equation until the difference between two calculated “Xc ” is lower than 0.01.
- Xi = Initial moisture content of the product when it is packaged (g g-1, d.b.).
- Kx = Permeability of the package to moisture vapor (kg H2O.m-2.Pa-1.day-1).
- Po = Vapor pressure at storage temperature (in Pa).
- A = Surface area of the package (m2).
- Ws = Weight of the product (kg of dry matter in the package).
- S = Slope of the product isotherm (assumed linear over the range between “Xe” and “Xc“).
Using the Heiss and Eichner model, we estimated the shelf-life of gari packaged in 1-kg polyethylene bags (A = 0.124 m2, Kx = 2.28 10-6 kg H2O.m-2.Pa-1.day-1) at three temperatures (15, 25, and 35 °C) and four initial moisture contents (6%, 8%, 10%, and 12% d.b.) (Table 2). As ambient storage conditions, we considered a relative humidity of 0.9 (which is the safe storage borderline humidity) and an aw of 0.7 (which is the safe storage borderline aw generally used for most products) (Pixton, 1982). Adeniji (1976) observed a significant growth of mould in gari stored at 27 °C, in a relative humidity of 0.7 and having an equilibrium content of 14.5% (d.b.). For aw 0.7, our sorption curves at 15, 25, and 35 °C give 14.0%, 14.2%, and 15.3% (d.b.) as equilibrium moisture contents (Table 2). These values agree with those of Adeniji (1976). Moisture contents around 14% (d.b.) allow gari to maintain a crispness that consumers greatly appreciate (Chuzel, 1989; Ekundayo, 1984).
Initial moisture content (d.b.) | Equilibration temperature (°C)a | ||
6 | 15 (14.0) | 25 (14.2) | 35 (15.3) |
6 | 181 | 90 | 57 |
8 | 166 | 85 | 52 |
10 | 140 | 73 | 45 |
12 | 89 | 24 | 30 |
a. Values in parentheses are equilibrium moisture contents (d.b.) for aw 0.7 (from experimental adsorption
isotherms).
Moisture contents before packaging (Xi ) are those generally found in local gari sold in tropical markets. lkediobi and Onyike (1982) mention moisture contents ranging from 4% to 19% (d.b.). The crossing-over of adsorption isotherms described above leads to the following paradox: gari stored at 35 °C seems to be better than that stored at 25 °C for a moisture content of 12% (d.b.). This moisture content is usually obtained with traditional gari processing (Chuzel, 1989), although storage time was less than 1 month under the given conditions.
We also focused on the permeability of packaging materials. Polyethylene, especially the high-density type, tends to inhibit water vapor transfer, but is permeable to oxygen and carbon dioxide, which may oxidize gari or cause loss of its aromas. Polypropylene is less permeable to water vapor and oxygen, but, because it is more expensive than polyethylene, it is less suitable for storing gari as a daily foodstuff for low-income consumers.
Conclusions
Gari processing modifies the structure of native cassava starch so that, at high water activity, it becomes more hygroscopic as temperature increases. Our study pointed out a “collapse” (not yet observed in other starchy products), caused by the partial gelatinization of gari starch. The GAB regression equation was adequate for fitting sorption isotherms of gari.
The shelf-life of gari was theoretically estimated for three storage temperatures (15, 25, and 35 °C) at a relative humidity of 0.9 (which is usual in the tropics). For a low-cost storage of at least 3 months at about 30 °C, we recommend packaging gari at an initial moisture content of about 8% (d.b.) in polyethylene bags. The bags should be sealed and tightly packed in cardboard boxes for both physical convenience and to prevent oxidation and reaction to light.
Further research should be carried out to determine the safe storage water activity in the tropics, taking into account the initial microbial flora of cassava roots and the local quality requirements for gari quality, that is, color, crispness, flavor, and food customs.