Intrinsic factors influencing the chemical modification of starch include the type of starch source (e.g. maize, potato, wheat), the botanical origin, granule size and shape, amylose to amylopectin ratio, and presence of impurities.
Extrinsic factors that influence chemical modification include processing conditions such as temperature, pH, and pressure, as well as the presence of other ingredients or contaminants in the system. Additionally, the choice of chemical modifying agent and the conditions of the modification reaction (e.g. time, temperature, concentration) can also impact the final modified starch structure and functionality.
Intrinsic factors
The granule architecture exhibits a marked effect on both starch molecular and granular patterns of reaction. One of the more compelling arguments in support of this notion is based on the differential molecular reaction patterns observed for granular and nongranular starches subjected to identical derivatization conditions (Steeneken and Smith, 1991; Jane et al., 1992; Steeneken and Woortman, 1994; Wilke and Mischnik, 1997; Richardson et al., 2003). For nongranular reactions, derivatization patterns on starch macromolecules are largely homogeneous or random in nature. In contrast, substituent groups on starch polymers modified in granular form are unevenly distributed among starch macromolecules and over their length; both observations have led to the hypothesis that molecular reaction sites within granular starch occur in clusters or blocks (Hood and Mercier, 1978; Steeneken and Smith, 1991; Steeneken and Woortman, 1994; Kavitha and BeMiller, 1998; Shi and BeMiller, 2000; Chen, Schols, and Voragen, 2004a).
For granular reactions, this uneven or heterogeneous molecular derivatization pattern is derived from the reality that reaction is preferentially and almost exclusively confined to granule amorphous regions (Hood and Mercier, 1978; Steeneken and Smith, 1991; Manelius et al., 2000a) and to the outer lamellae of crystalline regions (Chen, Schols, and Voragen, 2004). In this scenario, the amorphous regions of the granule, which generally contain AM molecules, branching regions of AP, and some nonreducing ends of AP branch chains are the favored loci for reaction (Hood and Mercier, 1978; Steeneken and Woortman, 1994; Kavitha and BeMiller, 1998). For most granular starch derivatives, AM is on average more heavily derivatized than AP (Steeneken and Woortman, 1994; Kavitha and BeMiller, 1998; Shi and BeMiller, 2000; Chen, Schols, and Voragen, 2004; Kaur, Singh, and Singh, 2004), although the reverse has also been reported (Salomonsson, Fransson, and Theander, 1991). Molecular reaction patterns of wheat, potato, and cassava starches (derivatized with a fluorescent probe) directly revealed the relative reaction densities for intact starch macromolecules (AM, AP) and AP branch chains (Figure 1) (Higley, 2005). Medium-length chains of AP (B1 and A chains) were least reacted, most likely due to their extensive involvement in the granule crystalline structure. Others have observed more than 40% of AP branch chains of DP ≈ 15 to be completely unreacted for the same reason cited above (Hood and Mercier, 1978; Kavitha and BeMiller, 1998). Reaction densities of long (B2, B3, and possibly some C chains) and very short AP branch chains (possibly too short for participation in the crystalline structure) were more than threefold higher than those of medium branch chains, and were equivalent to or greater than those of AM (Higley, 2005).
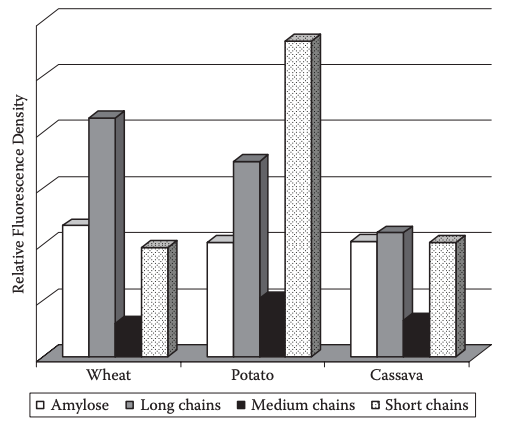
Although starches of various botanical sources share some fundamental similarities, it is essential to recognize that they differ in the specific details of their compositions and fine structures (AM:AP ratios, molecular structures, nonstarch constituents, etc.) and that these differences affect granular structure and, subsequently, reactivity. Heterogeneity among the various botanical sources and genotypes with respect to starch granule composition results in differential starch granule reactivities and reaction patterns (Mussulman and Wagoner, 1968; Singh, Ali, and Divakar, 1993; Azemi and Wootton, 1995; Bhattacharya, Singhal, and Kulkarni, 1995; Huber and BeMiller, 2001; Bertolini et al., 2003; Gray and BeMiller, 2005). In short, it is virtually certain that the granule structure affects both starch granular (crystalline vs. amorphous regions) and molecular reaction patterns, and that variation in structure, and thus reactivity, occurs not only among differing botantical sources of starch, but also within a given population of granules of a single source (Allen, Hood, and Parthasarathy, 1976; Ji et al., 2004).
Granule microstructure has also been shown to affect starch granule reactivity. Pores at the surfaces of corn, sorghum, millet, wheat, rye, and barley starch granules (Hall and Sayre, 1970; Fannon, Hauber, and BeMiller, 1992; Fannon, Schull, and BeMiller, 1993; Li et al., 2004; Waduge et al., 2006; Kim and Huber, 2008) were demonstrated to be openings to channels leading to the granule interior (Fannon, Schull, and BeMiller, 1993; Huber and BeMiller, 1997; Kim and Huber, 2008). Their characterization and influence on chemical modifications have been reviewed elsewhere (Fannon et al., 2003, 2004; Han et al., 2006a). For granules possessing channels, it was observed that the diffusion of dye (simulation of reagent flow) or reagent solution into the granule matrix occurred most readily through channels (laterally) and interior cavities (from the granule interior cavity outward into the matrix) (Huber and BeMiller, 2000, 2001). More recently, channels of corn and wheat starch granules are reported to be lined with protein (Han et al., 2005), which could further affect the reactivity or permeability of starch granules. In the absence of channels, reagent may enter the granule matrix via external granule surfaces (BeMiller, 1997), although, in the case of corn and sorghum starch granules, the rate of reagent entry by this mode appeared to be somewhat impeded by an outer shell, probably composed of tightly packed molecules (Huber and BeMiller, 2001). Consequently, for granules possessing pores and channels, these features facilitate reagent delivery into the granule matrix and significantly affect granular reaction patterns (Huber and BeMiller, 2001; Gray and BeMiller, 2004).
Starch derivatization is also reportedly influenced by starch granule size (Boruch, 1985; Bertolini et al., 2003; Stapley and BeMiller, 2003; Chen, Schols, and Voragen, 2004; Singh and Kaur, 2004; Van Hung and Morita, 2005a,b), although this effect has not been consistently observed in all cases (van der Burgt et al., 2000; Bertolini et al., 2003; Stapley and BeMiller, 2003). Small granules contribute a greater collective surface area for reaction on an equal starch weight basis when compared with large ones; however, the extent of starch derivatization does not always coincide with specific surface area measures. This observation is likely explained by the fact that differences in granule size are almost always confounded by other accompanying compositional or structural differences that themselves may also have an impact on granule reactivity. Thus, the effect of granule size is not always clear-cut, and can be reagent-specific (Bertolini et al., 2003).
Nonstarch constituents present in granules can also affect starch granule reactions. For example, waxy wheat starch was observed to exhibit a higher apparent reactivity relative to normal wheat starch in reactions with phosphoryl chloride (Bertolini et al., 2003). This observation is likely explained by the lack of a significant AM–lipid complex and a higher swelling power for waxy relative to normal wheat starch (Van Hung, Maeda, and Morita, 2007). The specific impact of AM–lipid complexes on granule reactivity has not been fully investigated, although Gunaratne and Corke (2007a) reported that these complexes are at least partially disrupted by alkaline conditions typical of starch reactions. In any event, the presence and stability of AM–lipid complexes have the potential to influence molecular mobility and reactivity of the granule undeveloped regions during reaction. Additionally, proteins are also anticipated substrates in starch reactions, due to their broad distribution at granule surfaces, inside channels (i.e., channel-associated), and within the granule matrix (i.e., granule-bound), and based on the fact that they contain nucleophilic groups capable of reacting with starch derivatizing reagents. The likelihood for protein to be located in accessible or amorphous regions of the granule further increases its potential for reaction. The potential accessibility and reactivity of the various starch granule proteins to a fluorescent probe have been demonstrated (Han and Hamaker, 2002; Han et al., 2005), although protein has been rarely, if at all, investigated as a factor in starch reactions involving industrial reagents.
Extrinsic factors
Of the various extrinsic factors, Gray and BeMiller (2005) identified pH as the most significant factor influencing starch reaction efficiency in the reaction with propylene oxide. For the majority of chemical modification schemes in which starch functions as a nucleophile, at least some degree of starch hydroxyl group ionization (formation of the alkoxide anion) is essential to “activate” starch for reaction. With a pKa ~12.6, starch hydroxyl groups would be expected to become increasingly ionized with an escalating pH. In general, an increased pH results in higher reaction efficiency (Wu and Seib, 1990; Lim and Seib, 1993; van Warners, Stamhuis, and Beenakers, 1994; Gray and BeMiller, 2005; Han and BeMiller, 2006), which is attributed to increased granule swelling (due to electrostatic repulsion between ionized starch hydroxyl groups) and/or increased starch alkoxide ion levels (BeMiller and Pratt, 1981; Gray and BeMiller, 2005). However, the effect of pH can vary with reagent type. An exception to the aforementioned trend may occur when a highly anionic reagent (e.g., sodium tripolyphosphate) becomes excessively ionized under alkaline conditions, inducing electrostatic repulsion between starch and reagent molecules to reduce the extent of reaction (Lim and Seib, 1993). In such cases, moderation of the reaction pH results in improved reaction efficiency.
The nature of the reaction medium greatly influences starch derivatization. Swelling–inhibiting salts (e.g., sodium sulfate, sodium chloride), which are frequently incorporated into the reaction medium to control granule swelling and prevent starch pasting, influence both the extent of derivatization (Shi and BeMiller, 2000; Villwock and BeMiller, 2005) and granular patterns of reaction (Gray and BeMiller, 2005). Approximately 1.8 times as much reagent was needed to achieve the same MS level on corn starch when a highly effective granule swelling inhibitor (potassium citrate) was used in the reaction medium in place of the usual sodium sulfate (Shi and BeMiller, 2000). Both the type and concentration of swelling-inhibiting salt are important considerations, as reaction medium conditions that promote a greater degree of granule swelling generally increase reagent access to the granule and the overall extent of derivatization (Shi and BeMiller, 2000; Gray and BeMiller, 2005; Villwock and BeMiller, 2005). Kweon, Bhirud, and Sosulski (1996), using an aqueous ethanol reaction system, observed that the extent of reaction was enhanced as the proportion of water in the reaction medium increased. In other cases, an increased ionic strength has been observed to enhance derivatization efficiency for reactions involving anionic reagents or reagent intermediates (Wu and Seib, 1990), possibly by shielding like charges between reagents and starch alkoxide anions (Woo and Seib, 1997).
For starch reacted as a granular slurry, the temperature of the reaction medium can be increased to enhance reactivity (Wu and Seib, 1990; Lammers, Stamhuis, and Beenackers, 1993; Woo and Seib, 1997), although temperature increases are generally limited to the starch sub gelatinization range (typically ≤50°C) to prevent pasting of granules. Possible explanations for reactivity enhancement with increasing temperature include:
- a decrease in the pKa of starch hydroxyl groups corresponding to an increased starch alkoxide ion concentration (Lammers, Stamhuis, and Beenackers, 1993),
- a reduction of the Donnan potential to grant catalyzing hydroxide anions greater access to starch granules (Oosten, 1982), or
- increased granule swelling due to electrostatic repulsion among neighboring starch alkoxide anions (Donovan, 1979; Gray and BeMiller, 2005).
Nevertheless, reactivity enhancement with increasing temperature has its limits. Wu and Seib (1990) reported that temperature was not a factor in cross-linking reactions conducted at pH values above 10.75, and Gray and BeMiller (2005) observed no significant temperature effect over the range of 44–54°C for substitution reactions. It would seem that once a specific degree of granule swelling or alkoxide level is achieved (via elevated pH or temperature), additional temperature increases offer no further benefit. Aside from starch–slurry reactions noted above, high temperature (120–170°C) is also used to drive derivatization of starch impregnated with inorganic (e.g., orthophosphate) salts using a semi-dry reaction process (Kerr and Cleveland, 1962). The heating of starch at reduced levels of moisture prevents the gelatinization of starch granules.
Reagent type affects starch granular reaction patterns. Fast-acting reagents (e.g., phosphoryl chloride) have been shown to be more likely to react at granule surfaces (first point of granular contact) to produce heterogeneous reaction patterns (Gluck-Hirsch and Kokini, 1997; Huber and BeMiller, 2001; Gray and BeMiller, 2004). In contrast, slow-reacting reagents generally produce more homogeneous granular reaction patterns, as the reagent has a longer timeframe to diffuse into and disperse throughout the granule matrix prior to reacting (Huber and BeMiller, 2001; Gray and BeMiller, 2004). Furthermore, the order of reagent addition during preparation of dual-modified starches affects overall derivatization levels and reaction patterns (Yeh and Yeh, 1993; Wang and Wang, 2000), implying that various reagents impart differential effects on granule reactivity. Initial cross-linking reduces the consequent extent of substitution, whereas initial substitution actually increases the extent of subsequent cross-linking (Yeh and Yeh, 1993). This finding is congruent with the general belief that the reagent reacts with the less-ordered (more amorphous) areas of granules first, causing these regions to swell. The swelling not only allows the amorphous regions to react more, but may also disrupt some of the crystalline regions, increasing the starch available for reaction (Gray and BeMiller, 2005). However, this general belief was not corroborated in starch reactions with propylene oxide (Han and BeMiller, 2005). It could be that this phenomenon is reagent-specific, as it is known to be possible to substitute starch granules (especially with anionic reagents) to such high levels that ambient temperature pasting of granules occurs as a function of extensive derivatization.
Although the appropriate length of reaction varies according to the reagent, most granular slurry reactions are thought to experience an initial stage of relatively high (reagent-specific) rate of reaction, in which the most accessible granular sites (amorphous regions) are reacted by reagent, followed by a decline or leveling off in reaction rate as the principal reaction sites become derivatized or reagent concentration declines (Han and BeMiller, 2005). The length of reaction can also potentially alter granule composition. For starch substitution reactions with propylene oxide, an increasing degree of leaching of starch material from granules was observed as the length of reaction increased (0–30 h). Molar substitution values for leached starch increased over the course of the reaction, and consistently exceeded those of the corresponding granular starch for all evaluated time intervals within the reaction period.